Just as our current understanding of the known universe comes to light, more inexplicable phenomenon has reached our eyes and minds with the advancement of technology and theoretical questioning. It was Galilei Galileo who in 1610 (Sidereus Nuncius) obtained enough resolution with his modified telescope design to see that the moon was rocky, rough and uneven, similar to our Earth as opposed to perfectly spherical and heavenly (a belief of Aristotelian cosmology). He also states that our own Milky Way and other nebulas (called such at the time) instead of being cloud-like were composed of many stars that we cannot see individually with our own eyes. Three hundred years later, mankind’s quench for understanding rose thanks to better observational equipment. Edwin Hubble proposed in 1919 with the help of the newly built Hooker Telescope and Cepheid Variables as a standard candle measure of distance, that nebulae in the sky were too far to be part of our Milky Way Galaxy: the only known galaxy at the time. Furthermore, they were galaxies themselves! There was much to learn about these newfound galaxies at the time. Many questions arose such as “How far are they? What bounds them together? How fast do they move? , etc”. These were some of the questions that astronomers strived to answer. Jan Oort in 1932 measured the orbital velocities of stars within our Galaxy and found that they orbited our galactic center faster than they should. Kepler’s laws and Newtonian Mechanics worked so beautifully to explain our solar system’s orbital speeds and the orbits of binary stars but failed to work on a galactic level. There had to be enough mass within our galaxy to account for the central gravity that held our Galaxy together. Oort found that the sum of visible matter such as stars within our galaxy was nowhere near the needed total mass to keep the Milky Way together. In other words, there was missing matter that was unavailable to our observations. In 1933, Fritz Zwicky, a Swiss astronomer studied the Coma cluster of galaxies. Zwicky believed that galaxies were bound together in clusters by gravity. This essentially enabled him to calculate an estimated mass of a said galaxy within the Coma Cluster based on both its average radial velocity and gravity alone. There was one problem though. Measurements of total mass based on gravity did not match the estimated total mass based on the sum of luminous material within the galaxy. In fact, it was 400 times greater than previously estimated. What held these galaxies together in the cluster? What enormous amount of mass was responsible for keeping such fast orbits around each other instead of careening away? There had to be some form of matter that was invisible and irresponsive to EM waves. This missing mass within our universe is not something directly observed. In fact, it could only be inferred by its indirect gravitational effects on other matter. Zwicky dubbed this, “Dark Matter”.
It was not until the 1970’s that the need of understanding dark matter was necessary. The 30’s were a time when the understanding of our cosmos was quite young. Einstein’s theories were just being tested and nuclear physics was a new field. By the 70’s, more advanced studies promised further evidence of Dark Matter’s existence. Newer instruments and computers along with refined theories of general relativity and the Big Bang brought this new unknown study into the forefront.
In the 70’s, American astronomer Vera Rubin studied the velocities of interstellar matter within luminous spiral galaxies. Rubin and her teammate Kent Ford at the Carnegie Institute in Washington used the Doppler Effect and the spectra of matter in galaxies to determine their orbital speeds around their galactic centers. Since most of the visible stars and dust are found near the center of a galaxy, Rubin and other astronomers at the time presumed that most of a galaxy’s mass and therefore it’s gravity lies in the center. Thinking this, they thought it was clear that the outermost stars and dust at the edges of galaxies should have lower velocities than those near the center (just like our own Solar System). This wasn’t the case when Rubin and Ford discovered that (through measuring the Doppler shift of H-alpha emission lines) the orbital velocities of outer emission regions in the neighboring Andromeda galaxy remained almost constant. Like Oort and Zwicky, Rubin was lead to believe that there was additional matter present in and around the Andromeda galaxy and some 60 others with similar observations that could account for those constant velocities as well as holding the galaxy together. Rubin put forth that the visible part of galaxies are only 1/10 the total mass of a galaxy and is also fixed within a halo consisting of dark matter extending far beyond the visible limit.
Understanding that dark matter cannot be seen though its existence is inferred, scientists were left to ask themselves, “What exactly could dark matter be composed of?” Knowing that it is non-luminous or does not emits EM waves; scientists thought that maybe dark matter was indeed normal invisible matter in the halo. This meant that dark matter could be anything from rogue planets with no parent stars to brown and white dwarfs or even neutron stars or black holes lying in the galactic halo. These objects were given the name MACHOs (Massive Astrophysical Compact Halo Objects) when teams of scientists in the 90s found evidence for baryonic (made of neutrons, neutron and thus atoms) dark matter in the form of stellar sized lumps in the Large Magellanic Cloud thanks to a phenomenon known as gravitational microlensing. Perhaps MACHOs could be an answer for the missing mass in our galaxy but not a valid one on a galactic level. All in all it would take many times more MACHOs to account for the missing mass and have any collective gravitational impact. Furthermore, dim lit white dwarfs have been favored as the prime candidate but scientists are not finding any detectable chemicals or elements of the stellar evolution leading into the white dwarf phase inside the halo.
Gravitational lensing is the deflection of light around objects of high mass. Predicted in Albert Einstein’s Theory of General Relativity, he states that the gravity of high mass objects can curve the spacetime around it. With this curve and gravity, a wave of photons in the form of visible light is bent accordingly. In one example, an observer looking directly at a far away galaxy will see its image undisturbed. Suppose now if there were to be a nearby galaxy cluster in between these two points. The light of our far out galaxy will be deflected around the gravitational force of the foreground cluster, creating a smeared, arced or distorted image of the background galaxy. The degree of lensing depends on the mass of the foreground object. In the case of the MACHO scientists in the 1990’s, MACHOs were detected via Gravitational Microlensing. Which is a phenomenon perfect for smaller, lesser mass objects. In this case, the lensing effect is so minor that it doesn’t distort or sheer the image. Rather, it redirects and bends missing light from a background star that otherwise would have missed us back towards our field of view. This causes an increased flux of the background star, making it seem brighter while the MACHO is transient. This shift in brightness is hard to observe and is thus detected photometrically by computers. Unfortunately, multiple teams of scientists have not found sufficient evidence of enough MACHOs by this method. MACHOs must not be responsible for the missing mass of the universe.
The definitive arcing and smearing of background objects through strong gravitational lensing is now a method of detection for dark matter. Dark matter is invisible to us and because dark matter only reacts with the gravitational force as opposed to the electromagnetic force, we can infer its presence by its apparent lensing effect. Dense patches of otherwise invisible dark matter can now be detected by the distortions of background galaxies and clusters behind it as if nothing is in between them and our eyes.
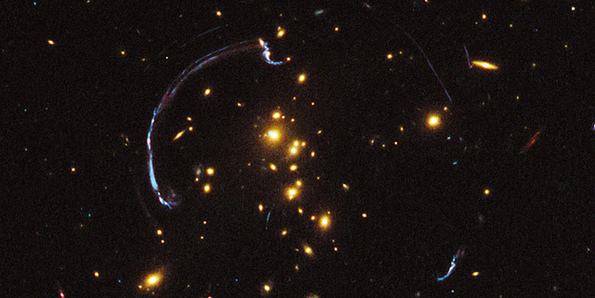
Light from this galaxy is bent into a full 90 degree arc due to gravitational lensing.
Image courtesy NASA/ESA/J. Rigby/K. Sharon.
We can use the amount of lensing along with the angle of bending to properly take a survey of dark matter and map out the structure of our universe as a whole. It is now possible to map out the whereabouts and distribution of dark matter in detail on an intergalactic scale. Using data from the Hubble Space Telescope, astronomers in 2007 were able to create a 3D model of the distribution of dark matter after analysis of the Hubble COSMOS Survey. Astronomers found that the dark matter extended out in a network of filaments and met at huge densities of galactic clusters. This showed how galaxies assemble under the high densities of dark matter shedding light into galaxy formation and the shape of the early universe.
The study of dark matter is one of physics and astronomy’s newest challenges. Being on the edge of our current knowledge, dark matter might be something new and unstudied. Leading theorists claim that since baryonic matter accounts for only 4% of the universe, dark matter must be a non-baryonic subatomic particle. In the 80s, it was presumed that neutrinos, which travel near the speed of light and have very small mass could be the constituent of dark matter since it shares traits such as, no EM interaction and no interaction to other particles. This hypothesis though was put down as modeling of the early universe with neutrinos and their small mass, failed to explain the clumping of matters into stars and galaxies after the Big Bang. Neutrinos with their relatively very small mass and speed almost as fast as light pose as the wrong candidate for mass accumulation in the early universe. Scientists hypothesize a new undetected particle as being the best candidate for dark matter. Similar to neutrinos, a new particle would need to be much slower and many times more massive. It is hypothesized through evidence, that a new subatomic particle known as a WIMP (weakly interacting massive particle) and their response to gravity are responsible for the clumping of the universe that later formed into primordial galaxies. Currently there are no known particles that have all the traits of a WIMP therefore it is a leading hypothesis on both a new particle as well as the dark matter mystery. Both astronomers and particle physicists around the world are currently conducting massive research efforts in order to find this theorized particle. Particle detection is based on detecting a WIMPs interaction with an atomic nucleus such as that in the Soudan Mine by the Cryogenic Dark Matter Search team.
Perhaps WIMPs do not exist. Or maybe dark matter doesn’t exist at all. One very popular counter theory states that our current understanding of gravity described by Newtonian Theory, does not work on an intergalactic scale. That is, it is something we have not yet fully understood yet. Israeli physicist Mordehai Milgrom in 1983 proposed that a modification of Newtonian gravity would be needed to explain phenomena such as the constant orbital velocities in galaxies. This has been labeled as Modified Newtonian Dynamics or MOND. Since the Standard Model of cosmology works to well and accurately to explain the motions of everything else in our universe, it would be very difficult for the scientific community to say that this, along with our theories of gravity and relativity are incorrect.
The search for dark matter is in my opinion, is science’s greatest endeavor. The limits of our own understanding in all the history of physics is now once more looking to further its reach, far beyond the cosmos. What would it mean to mankind if we were to say that we aren’t even made up of the same stuff as the cosmos. To say that we are even more insignificant than we once thought we were. That even the stars and the visible galaxies in the whole of our universe still cannot account for even a quarter of all matter. Astronomers like Oort and Zwicky showed the world that the understanding of the cosmos was in our grasps. They led to influence Vera Rubin and her magnificent findings along with scientists around the world to solve physics’ greatest mystery. Powerful new machines, owing their own existence to modern physics can detect and see the effects of dark matter through gravitational lensing. Our understanding of that then allowed us to view distributions of this mysterious matter. Mankind as a whole is making strides together to figure out what it is that make up our heavens and perhaps, our own existence. Astronomers, astrophysicists and particle physicists from around the work have set forth this effort of demystifying dark matter and our universe. Whether you are on the side of the Standard Model or conceivably, Modified Newtonian Dynamics, it is clear that like all of science throughout history, past assumptions may need to be taken with a grain of salt when approaching new ideas. Vera Rubin said, “Science progresses best when observations force us to alter our preconceptions.” Today we are in a new place in science as well as a new place within the ever-expanding universe we once though we knew. Hopefully one day soon, some clear defining light can shine down on the mystery that is dark matter and that the next great physicist since Einstein be rewarded with the coveted Nobel Prize among others.

In this computer simulation, dark matter provides the cosmic structure of the universe we know today.
Works Cited
1) Panek, Richard. The 4 Percent Universe: Dark Matter, Dark Energy, and the Race to Discover the Rest of Reality. N.p.: Mariner Books, 2011.
2) Ostriker, Jeremiah P., and Paul Steinhardt. “New Light on Dark Matter.” Science, New Series 300.5627 (2003): 1909-913. JSTOR. Web. http://www.jstor.org/stable/3834518.
3) “Bending of Light May Help Scientists Find Remote Objects.” The Science News-Letter 31.826 (1937): 87. JSTOR. Web.
4) “The MOND Pages.” The MOND Pages. University of Maryland, n.d. Web. http://www.astro.umd.edu/~ssm/mond/.
5) “Dark Matter.” SuperCDMS at Queen’s University. Queens University, n.d. Web. http://cdms.phy.queensu.ca/.
6) “Vera Rubin and Dark Matter.” Vera Rubin and Dark Matter. UCLA, n.d. Web. http://www.physics.ucla.edu/~cwp/articles/rubindm/rubindm.html.