Contents
Hormones & Behavior
By Randy J. Nelson
The Ohio State University
The goal of this module is to introduce you to the topic of hormones and behavior. This field of study is also called behavioral endocrinology, which is the scientific study of the interaction between hormones and behavior. This interaction is bidirectional: hormones can influence behavior, and behavior can sometimes influence hormone concentrations. Hormones are chemical messengers released from endocrine glands that travel through the blood system to influence the nervous system to regulate behaviors such as aggression, mating, and parenting of individuals.
Learning Objectives
- Define the basic terminology and basic principles of hormone–behavior interactions.
- Explain the role of hormones in behavioral sex differentiation.
- Explain the role of hormones in aggressive behavior.
- Explain the role of hormones in parental behavior.
- Provide examples of some common hormone–behavior interactions.
Introduction
This module describes the relationship between hormones and behavior. Many readers are likely already familiar with the general idea that hormones can affect behavior. Students are generally familiar with the idea that sex-hormone concentrations increase in the blood during puberty and decrease as we age, especially after about 50 years of age. Sexual behavior shows a similar pattern. Most people also know about the relationship between aggression and anabolic steroid hormones, and they know that administration of artificial steroid hormones sometimes results in uncontrollable, violent behavior called “roid rage.” Many different hormones can influence several types of behavior, but for the purpose of this module, we will restrict our discussion to just a few examples of hormones and behaviors. For example, are behavioral sex differences the result of hormones, the environment, or some combination of factors? Why are men much more likely than women to commit aggressive acts? Are hormones involved in mediating the so-called maternal “instinct”? Behavioral endocrinologists are interested in how the general physiological effects of hormones alter the development and expression of behavior and how behavior may influence the effects of hormones. This module describes, both phenomenologically and functionally, how hormones affect behavior.
To understand the hormone-behavior relationship, it is important briefly to describe hormones. Hormones are organic chemical messengers produced and released by specialized glands called endocrine glands. Hormones are released from these glands into the blood, where they may travel to act on target structures at some distance from their origin. Hormones are similar in function to neurotransmitters, the chemicals used by the nervous system in coordinating animals’ activities. However, hormones can operate over a greater distance and over a much greater temporal range than neurotransmitters (Focus Topic 1). Examples of hormones that influence behavior include steroid hormones such as testosterone (a common type of androgen), estradiol (a common type of estrogen), progesterone (a common type of progestin), and cortisol (a common type of glucocorticoid) (Table 1, A-B). Several types of protein or peptide (small protein) hormones also influence behavior, including oxytocin, vasopressin, prolactin, and leptin.
Although neural and hormonal communication both rely on chemical signals, several prominent differences exist. Communication in the nervous system is analogous to traveling on a train. You can use the train in your travel plans as long as tracks exist between your proposed origin and destination. Likewise, neural messages can travel only to destinations along existing nerve tracts. Hormonal communication, on the other hand, is like traveling in a car. You can drive to many more destinations than train travel allows because there are many more roads than railroad tracks. Similarly, hormonal messages can travel anywhere in the body via the circulatory system; any cell receiving blood is potentially able to receive a hormonal message.
Neural and hormonal communication differ in other ways as well. To illustrate them, consider the differences between digital and analog technologies. Neural messages are digital, all-or-none events that have rapid onset and offset: neural signals can take place in milliseconds. Accordingly, the nervous system mediates changes in the body that are relatively rapid. For example, the nervous system regulates immediate food intake and directs body movement. In contrast, hormonal messages are analog, graded events that may take seconds, minutes, or even hours to occur. Hormones can mediate long-term processes, such as growth, development, reproduction, and metabolism.
Hormonal and neural messages are both chemical in nature, and they are released and received by cells in a similar manner; however, there are important differences as well. Neurotransmitters, the chemical messengers used by neurons, travel a distance of only 20–30 nanometers (30 X 10–9 m)—to the membrane of the postsynaptic neuron, where they bind with receptors. Hormones enter the circulatory system and may travel from 1 millimeter to >2 meters before arriving at a target cell, where they bind with specific receptors.
Another distinction between neural and hormonal communication is the degree of voluntary control that can be exerted over their functioning. In general, there is more voluntary control of neural than of hormonal signals. It is virtually impossible to will a change in your thyroid hormone levels, for example, whereas moving your limbs on command is easy.
Although these are significant differences, the division between the nervous system and the endocrine system is becoming more blurred as we learn more about how the nervous system regulates hormonal communication. A better understanding of the interface between the endocrine system and the nervous system, called neuroendocrinology, is likely to yield important advances in the future study of the interaction between hormones and behavior.
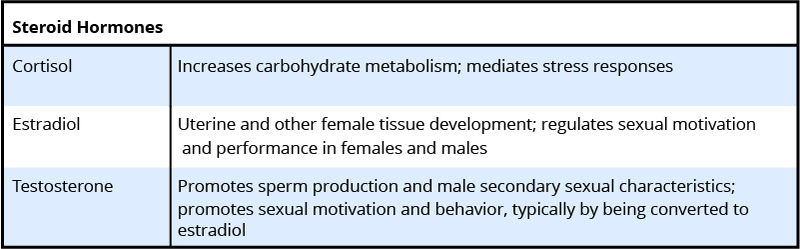
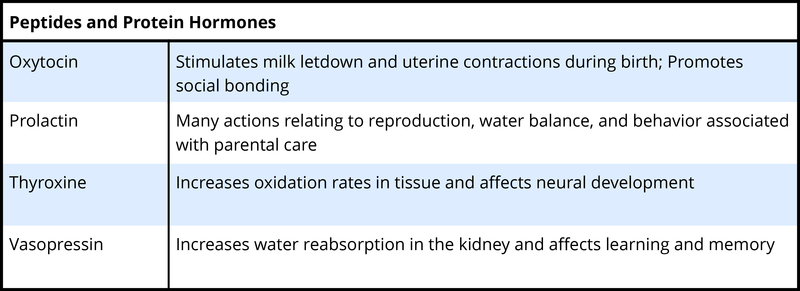
Hormones coordinate the physiology and behavior of individuals by regulating, integrating, and controlling bodily functions. Over evolutionary time, hormones have often been co-opted by the nervous system to influence behavior to ensure reproductive success. For example, the same hormones, testosterone and estradiol, that cause gamete (egg or sperm) maturation also promote mating behavior. This dual hormonal function ensures that mating behavior occurs when animals have mature gametes available for fertilization. Another example of endocrine regulation of physiological and behavioral function is provided by pregnancy. Estrogens and progesterone concentrations are elevated during pregnancy, and these hormones are often involved in mediating maternal behavior in the mothers.
Not all cells are influenced by each and every hormone. Rather, any given hormone can directly influence only cells that have specific hormone receptors for that particular hormone. Cells that have these specific receptors are called target cells for the hormone. The interaction of a hormone with its receptor begins a series of cellular events that eventually lead to activation of enzymatic pathways or, alternatively, turns on or turns off gene activation that regulates protein synthesis. The newly synthesized proteins may activate or deactivate other genes, causing yet another cascade of cellular events. Importantly, sufficient numbers of appropriate hormone receptors must be available for a specific hormone to produce any effects. For example, testosterone is important for male sexual behavior. If men have too little testosterone, then sexual motivation may be low, and it can be restored by testosterone treatment. However, if men have normal or even elevated levels of testosterone yet display low sexual drive, then it might be possible for a lack of receptors to be the cause and treatment with additional hormones will not be effective.
How might hormones affect behavior? In terms of their behavior, one can think of humans and other animals conceptually as comprised of three interacting components: (1) input systems (sensory systems), (2) integrators (the central nervous system), and (3) output systems, or effectors (e.g., muscles). Hormones do not causebehavioral changes. Rather, hormones influence these three systems so that specific stimuli are more likely to elicit certain responses in the appropriate behavioral or social context. In other words, hormones change the probability that a particular behavior will be emitted in the appropriate situation (Nelson, 2011). This is a critical distinction that can affect how we think of hormone-behavior relationships.
We can apply this three-component behavioral scheme to a simple behavior, singing in zebra finches. Only male zebra finches sing. If the testes of adult male finches are removed, then the birds reduce singing, but castrated finches resume singing if the testes are reimplanted, or if the birds are treated with either testosterone or estradiol. Although we commonly consider androgens to be “male” hormones and estrogens to be “female” hormones, it is common for testosterone to be converted to estradiol in nerve cells (Figure 1). Thus, many male-like behaviors are associated with the actions of estrogens! Indeed, all estrogens must first be converted from androgens because of the typical biochemical synthesis process. If the converting enzyme is low or missing, then it is possible for females to produce excessive androgens and subsequently develop associated male traits. It is also possible for estrogens in the environment to affect the nervous system of animals, including people (e.g., Kidd et al., 2007). Again, singing behavior is most frequent when blood testosterone or estrogen concentrations are high. Males sing to attract mates or ward off potential competitors from their territories.
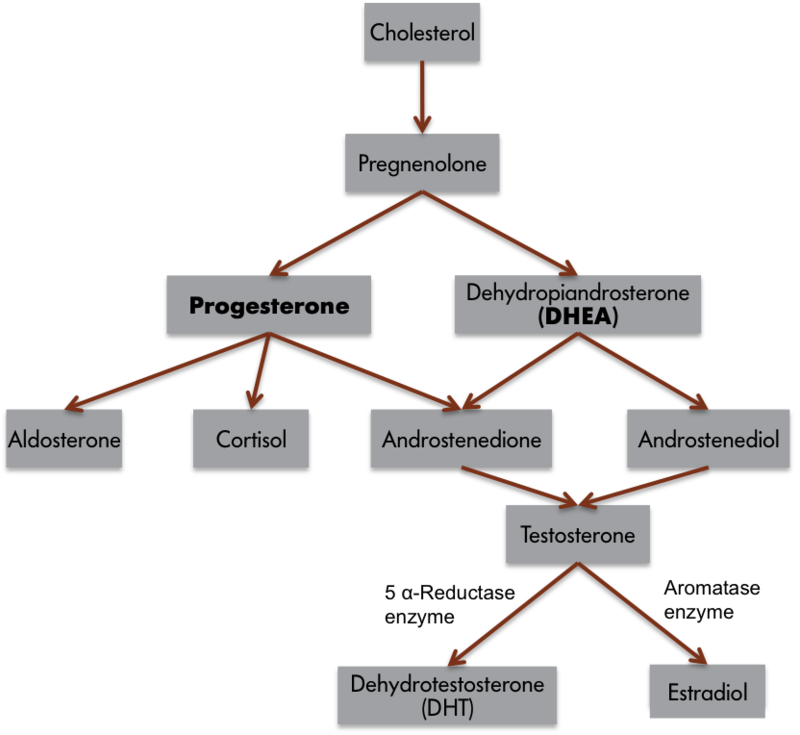
Although it is apparent from these observations that estrogens are somehow involved in singing, how might the three-component framework just introduced help us to formulate hypotheses to explore estrogen’s role in this behavior? By examining input systems, we could determine whether estrogens alter the birds’ sensory capabilities, making the environmental cues that normally elicit singing more salient. If this were the case, then females or competitors might be more easily seen or heard. Estrogens also could influence the central nervous system. Neuronal architecture or the speed of neural processing could change in the presence of estrogens. Higher neural processes (e.g., motivation, attention, or perception) also might be influenced. Finally, the effector organs, muscles in this case, could be affected by the presence of estrogens. Blood estrogen concentrations might somehow affect the muscles of a songbird’s syrinx (the vocal organ of birds). Estrogens, therefore, could affect birdsong by influencing the sensory capabilities, central processing system, or effector organs of an individual bird. We do not understand completely how estrogen, derived from testosterone, influences birdsong, but in most cases, hormones can be considered to affect behavior by influencing one, two, or all three of these components, and this three-part framework can aid in the design of hypotheses and experiments to explore these issues.
How might behaviors affect hormones? The birdsong example demonstrates how hormones can affect behavior, but as noted, the reciprocal relation also occurs; that is, behavior can affect hormone concentrations. For example, the sight of a territorial intruder may elevate blood testosterone concentrations in resident male birds and thereby stimulate singing or fighting behavior. Similarly, male mice or rhesus monkeys that lose a fight decrease circulating testosterone concentrations for several days or even weeks afterward. Comparable results have also been reported in humans. Testosterone concentrations are affected not only in humans involved in physical combat, but also in those involved in simulated battles. For example, testosterone concentrations were elevated in winners and reduced in losers of regional chess tournaments.
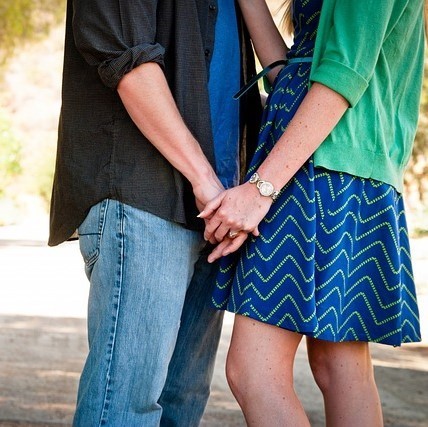
People do not have to be directly involved in a contest to have their hormones affected by the outcome of the contest. Male fans of both the Brazilian and Italian teams were recruited to provide saliva samples to be assayed for testosterone before and after the final game of the World Cup soccer match in 1994. Brazil and Italy were tied going into the final game, but Brazil won on a penalty kick at the last possible moment. The Brazilian fans were elated and the Italian fans were crestfallen. When the samples were assayed, 11 of 12 Brazilian fans who were sampled had increased testosterone concentrations, and 9 of 9 Italian fans had decreased testosterone concentrations, compared with pre-game baseline values (Dabbs, 2000).
In some cases, hormones can be affected by anticipation of behavior. For example, testosterone concentrations also influence sexual motivation and behavior in women. In one study, the interaction between sexual intercourse and testosterone was compared with other activities (cuddling or exercise) in women (van Anders, Hamilton, Schmidt, & Watson, 2007). On three separate occasions, women provided a pre-activity, post-activity, and next-morning saliva sample. After analysis, the women’s testosterone was determined to be elevated prior to intercourse as compared to other times. Thus, an anticipatory relationship exists between sexual behavior and testosterone. Testosterone values were higher post-intercourse compared to exercise, suggesting that engaging in sexual behavior may also influence hormone concentrations in women.
Sex Differences
Hens and roosters are different. Cows and bulls are different. Men and women are different. Even girls and boys are different. Humans, like many animals, are sexually dimorphic (di, “two”; morph, “type”) in the size and shape of their bodies, their physiology, and for our purposes, their behavior. The behavior of boys and girls differs in many ways. Girls generally excel in verbal abilities relative to boys; boys are nearly twice as likely as girls to suffer from dyslexia (reading difficulties) and stuttering and nearly 4 times more likely to suffer from autism. Boys are generally better than girls at tasks that require visuospatial abilities. Girls engage in nurturing behaviors more frequently than boys. More than 90% of all anorexia nervosa cases involve young women. Young men are twice as likely as young women to suffer from schizophrenia. Boys are much more aggressive and generally engage in more rough-and-tumble play than girls (Berenbaum, Martin, Hanish, Briggs, & Fabes, 2008). Many sex differences, such as the difference in aggressiveness, persist throughout adulthood. For example, there are many more men than women serving prison sentences for violent behavior. The hormonal differences between men and women may account for adult sex differences that develop during puberty, but what accounts for behavioral sex differences among children prior to puberty and activation of their gonads? Hormonal secretions from the developing gonads determine whether the individual develops in a male or female manner. The mammalian embryonic testes produce androgens, as well as peptide hormones, that steer the development of the body, central nervous system, and subsequent behavior in a male direction. The embryonic ovaries of mammals are virtually quiescent and do not secrete high concentrations of hormones. In the presence of ovaries, or in the complete absence of any gonads, morphological, neural, and, later, behavioral development follows a female pathway.
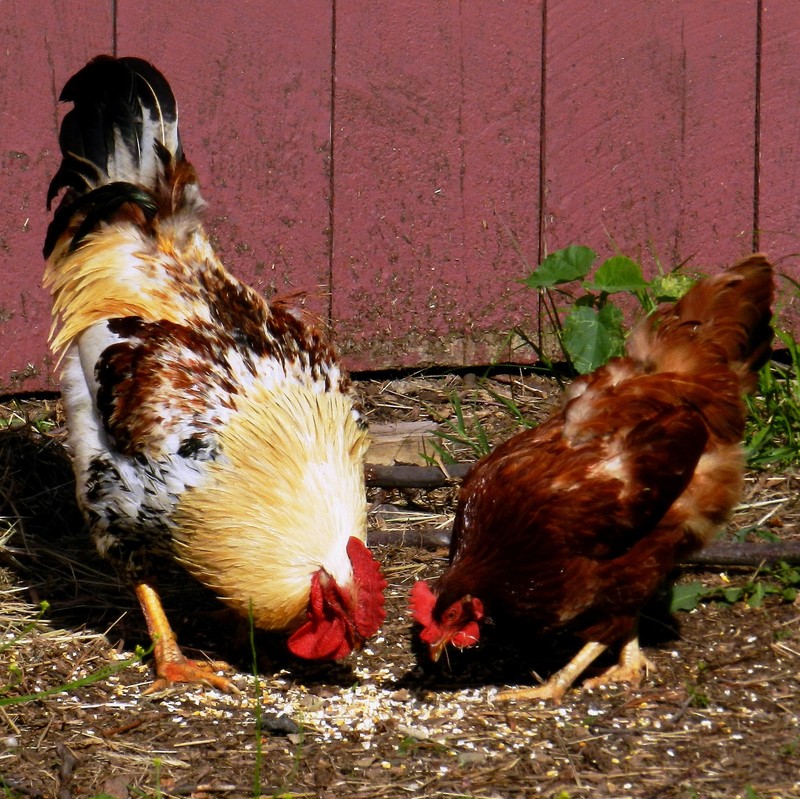
Gonadal steroid hormones have organizational (or programming) effects upon brain and behavior (Phoenix, Goy, Gerall, & Young, 1959). The organizing effects of steroid hormones are relatively constrained to the early stages of development. An asymmetry exists in the effects of testes and ovaries on the organization of behavior in mammals. Hormone exposure early in life has organizational effects on subsequent rodent behavior; early steroid hormone treatment causes relatively irreversible and permanent masculinization of rodent behavior (mating and aggressive). These early hormone effects can be contrasted with the reversible behavioral influences of steroid hormones provided in adulthood, which are called activational effects. The activational effects of hormones on adult behavior are temporary and may wane soon after the hormone is metabolized. Thus, typical male behavior requires exposure to androgens during gestation (in humans) or immediately after birth (in rodents) to somewhat masculinize the brain and also requires androgens during or after puberty to activate these neural circuits. Typical female behavior requires a lack of exposure to androgens early in life which leads to feminization of the brain and also requires estrogens to activate these neural circuits in adulthood. But this simple dichotomy, which works well with animals with very distinct sexual dimorphism in behavior, has many caveats when applied to people.
If you walk through any major toy store, then you will likely observe a couple of aisles filled with pink boxes and the complete absence of pink packaging of toys in adjacent aisles. Remarkably, you will also see a strong self-segregation of boys and girls in these aisles. It is rare to see boys in the “pink” aisles and vice versa. The toy manufacturers are often accused of making toys that are gender biased, but it seems more likely that boys and girls enjoy playing with specific types and colors of toys. Indeed, toy manufacturers would immediately double their sales if they could sell toys to both sexes. Boys generally prefer toys such as trucks and balls and girls generally prefer toys such as dolls. Although it is doubtful that there are genes that encode preferences for toy cars and trucks on the Y chromosome, it is possible that hormones might shape the development of a child’s brain to prefer certain types of toys or styles of play behavior. It is reasonable to believe that children learn which types of toys and which styles of play are appropriate to their gender. How can we understand and separate the contribution of physiological mechanisms from learning to understand sex differences in human behaviors? To untangle these issues, animal models are often used. Unlike the situation in humans, where sex differences are usually only a matter of degree (often slight), in some animals, members of only one sex may display a particular behavior. As noted, often only male songbirds sing. Studies of such strongly sex-biased behaviors are particularly valuable for understanding the interaction among behavior, hormones, and the nervous system.
A study of vervet monkeys calls into question the primacy of learning in the establishment of toy preferences (Alexander & Hines, 2002). Female vervet monkeys preferred girl-typical toys, such as dolls or cooking pots, whereas male vervet monkeys preferred boy-typical toys, such as cars or balls. There were no sex differences in preference for gender-neutral toys, such as picture books or stuffed animals. Presumably, monkeys have no prior concept of “boy” or “girl” toys. Young rhesus monkeys also show similar toy preferences.
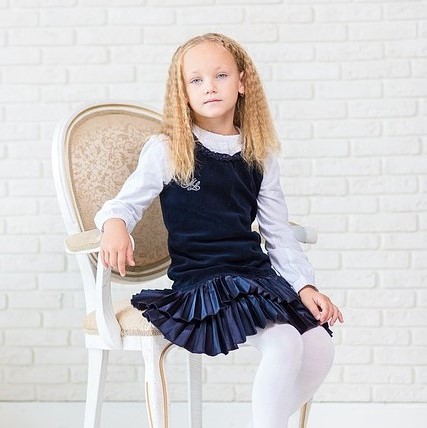
What then underlies the sex difference in toy preference? It is possible that certain attributes of toys (or objects) appeal to either boys or girls. Toys that appeal to boys or male vervet or rhesus monkeys, in this case, a ball or toy car, are objects that can be moved actively through space, toys that can be incorporated into active, rough and tumble play. The appeal of toys that girls or female vervet monkeys prefer appears to be based on color. Pink and red (the colors of the doll and pot) may provoke attention to infants.
Society may reinforce such stereotypical responses to gender-typical toys. The sex differences in toy preferences emerge by 12 or 24 months of age and seem fixed by 36 months of age, but are sex differences in toy preference present during the first year of life? It is difficult to ask pre-verbal infants what they prefer, but in studies where the investigators examined the amount of time that babies looked at different toys, eye-tracking data indicate that infants as young as 3 months showed sex differences in toy preferences; girls preferred dolls, whereas boys preferred trucks. Another result that suggests, but does not prove, that hormones are involved in toy preferences is the observation that girls diagnosed with congenital adrenal hyperplasia (CAH), whose adrenal glands produce varying amounts of androgens early in life, played with masculine toys more often than girls without CAH. Further, a dose-response relationship between the extent of the disorder (i.e., degree of fetal androgen exposure) and degree of masculinization of play behavior was observed. Are the sex differences in toy preferences or play activity, for example, the inevitable consequences of the differential endocrine environments of boys and girls, or are these differences imposed by cultural practices and beliefs? Are these differences the result of receiving gender-specific toys from an early age, or are these differences some combination of endocrine and cultural factors? Again, these are difficult questions to unravel in people.
Even when behavioral sex differences appear early in development, there seems to be some question regarding the influences of societal expectations. One example is the pattern of human play behavior during which males are more physical; this pattern is seen in a number of other species including nonhuman primates, rats, and dogs. Is the difference in the frequency of rough-and-tumble play between boys and girls due to biological factors associated with being male or female, or is it due to cultural expectations and learning? If there is a combination of biological and cultural influences mediating the frequency of rough-and-tumble play, then what proportion of the variation between the sexes is due to biological factors and what proportion is due to social influences? Importantly, is it appropriate to talk about “normal” sex differences when these traits virtually always arrange themselves along a continuum rather than in discrete categories?
Sex differences are common in humans and in nonhuman animals. Because males and females differ in the ratio of androgenic and estrogenic steroid hormone concentrations, behavioral endocrinologists have been particularly interested in the extent to which behavioral sex differences are mediated by hormones. The process of becoming female or male is called sexual differentiation. The primary step in sexual differentiation occurs at fertilization. In mammals, the ovum (which always contains an X chromosome) can be fertilized by a sperm bearing either a Y or an X chromosome; this process is called sex determination. The chromosomal sex of homogametic mammals (XX) is female; the chromosomal sex of heterogametic mammals (XY) is male. Chromosomal sex determines gonadal sex. Virtually all subsequent sexual differentiation is typically the result of differential exposure to gonadal steroid hormones. Thus, gonadal sex determines hormonal sex, which regulates morphological sex. Morphological differences in the central nervous system, as well as in some effector organs, such as muscles, lead to behavioral sex differences. The process of sexual differentiation is complicated, and the potential for errors is present. Perinatal exposure to androgens is the most common cause of anomalous sexual differentiation among females. The source of androgen may be internal (e.g., secreted by the adrenal glands) or external (e.g., exposure to environmental estrogens). Turner syndrome results when the second X chromosome is missing or damaged; these individuals possess dysgenic ovaries and are not exposed to steroid hormones until puberty. Interestingly, women with Turner syndrome often have impaired spatial memory.
Female mammals are considered the “neutral” sex; additional physiological steps are required for male differentiation, and more steps bring more possibilities for errors in differentiation. Some examples of male anomalous sexual differentiation include 5α-reductase deficiency (in which XY individuals are born with ambiguous genitalia because of a lack of dihydrotestosterone and are reared as females, but masculinization occurs during puberty) and androgen insensitivity syndrome or TFM (in which XY individuals lack receptors for androgens and develop as females). By studying individuals who do not neatly fall into the dichotic boxes of female or male and for whom the process of sexual differentiation is atypical, behavioral endocrinologists glean hints about the process of typical sexual differentiation.
We may ultimately want to know how hormones mediate sex differences in the human brain and behavior (to the extent to which these differences occur). To understand the mechanisms underlying sex differences in the brain and behavior, we return to the birdsong example. Birds provide the best evidence that behavioral sex differences are the result of hormonally induced structural changes in the brain (Goodson, Saldanha, Hahn, & Soma, 2005). In contrast to mammals, in which structural differences in neural tissues have not been directly linked to behavior, structural differences in avian brains have been directly linked to a sexually behavior: birdsong.
Several brain regions in songbirds display significant sex differences in size. Two major brain circuit pathways, (1) the song production motor pathway and (2) the auditory transmission pathway, have been implicated in the learning and production of birdsong. Some parts of the song production pathway of male zebra finches are 3 to 6 times larger than those of female conspecifics. The larger size of these brain areas reflects that neurons in these nuclei are larger, more numerous, and farther apart. Although castration of adult male birds reduces singing, it does not reduce the size of the brain nuclei controlling song production. Similarly, androgen treatment of adult female zebra finches does not induce changes either in singing or in the size of the song control regions. Thus, activational effects of steroid hormones do not account for the sex differences in singing behavior or brain nucleus size in zebra finches. The sex differences in these structures are organized or programmed in the egg by estradiol (masculinizes) or the lack of steroids (feminizes).
Taken together, estrogens appear to be necessary to activate the neural machinery underlying the song system in birds. The testes of birds primarily produce androgens, which enter the circulation. The androgens enter neurons containing aromatase, which converts them to estrogens. Indeed, the brain is the primary source of estrogens, which activate masculine behaviors in many bird species.
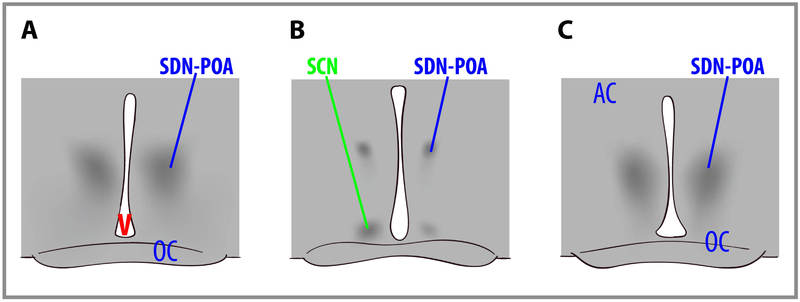
Sex differences in human brain size have been reported for years. More recently, sex differences in specific brain structures have been discovered (Figure 2). Sex differences in a number of cognitive functions have also been reported. Females are generally more sensitive to auditory information, whereas males are more sensitive to visual information. Females are also typically more sensitive than males to taste and olfactory input. Women display less lateralization of cognitive functions than men. On average, females generally excel in verbal, perceptual, and fine motor skills, whereas males outperform females on quantitative and visuospatial tasks, including map reading and direction finding. Although reliable sex differences can be documented, these differences in ability are slight. It is important to note that there is more variation within each sex than betweenthe sexes for most cognitive abilities (Figure 3).
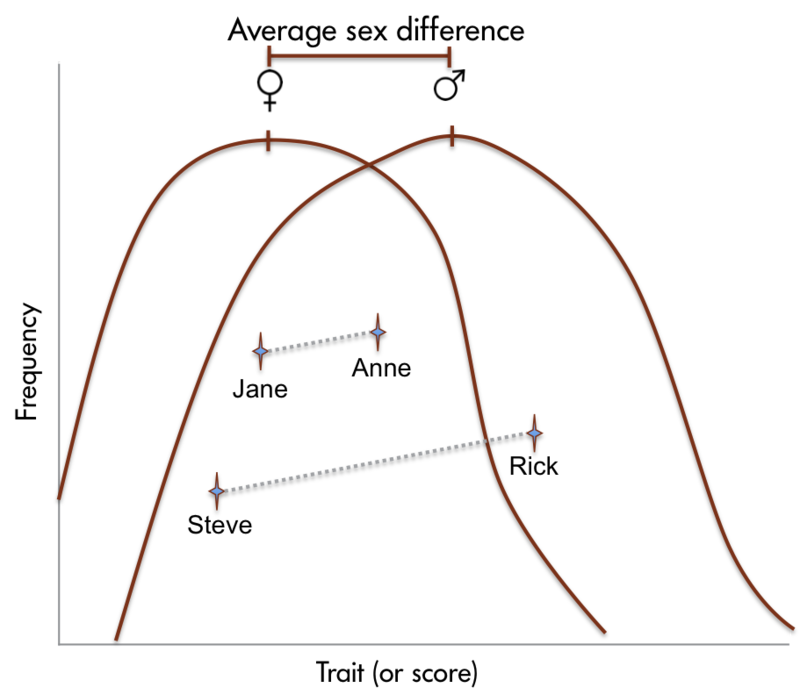
Aggressive Behaviors
The possibility for aggressive behavior exists whenever the interests of two or more individuals are in conflict (Nelson, 2006). Conflicts are most likely to arise over limited resources such as territories, food, and mates. A social interaction decides which animal gains access to the contested resource. In many cases, a submissive posture or gesture on the part of one animal avoids the necessity of actual combat over a resource. Animals may also participate in threat displays or ritualized combat in which dominance is determined but no physical damage is inflicted.
There is overwhelming circumstantial evidence that androgenic steroid hormones mediate aggressive behavior across many species. First, seasonal variations in blood plasma concentrations of testosterone and seasonal variations in aggression coincide. For instance, the incidence of aggressive behavior peaks for male deer in autumn, when they are secreting high levels of testosterone. Second, aggressive behaviors increase at the time of puberty, when the testes become active and blood concentrations of androgens rise. Juvenile deer do not participate in the fighting during the mating season. Third, in any given species, males are generally more aggressive than females. This is certainly true of deer; relative to stags, female deer rarely display aggressive behavior, and their rare aggressive acts are qualitatively different from the aggressive behavior of aggressive males. Finally, castration typically reduces aggression in males, and testosterone replacement therapy restores aggression to pre-castration levels. There are some interesting exceptions to these general observations that are outside the scope of this module.
As mentioned, males are generally more aggressive than females. Certainly, human males are much more aggressive than females. Many more men than women are convicted of violent crimes in North America. The sex differences in human aggressiveness appear very early. At every age throughout the school years, many more boys than girls initiate physical assaults. Almost everyone will acknowledge the existence of this sex difference, but assigning a cause to behavioral sex differences in humans always elicits much debate. It is possible that boys are more aggressive than girls because androgens promote aggressive behavior and boys have higher blood concentrations of androgens than girls. It is possible that boys and girls differ in their aggressiveness because the brains of boys are exposed to androgens prenatally and the “wiring” of their brains is thus organized in a way that facilitates the expression of aggression. It is also possible that boys are encouraged and girls are discouraged by family, peers, or others from acting in an aggressive manner. These three hypotheses are not mutually exclusive, but it is extremely difficult to discriminate among them to account for sex differences in human aggressiveness.
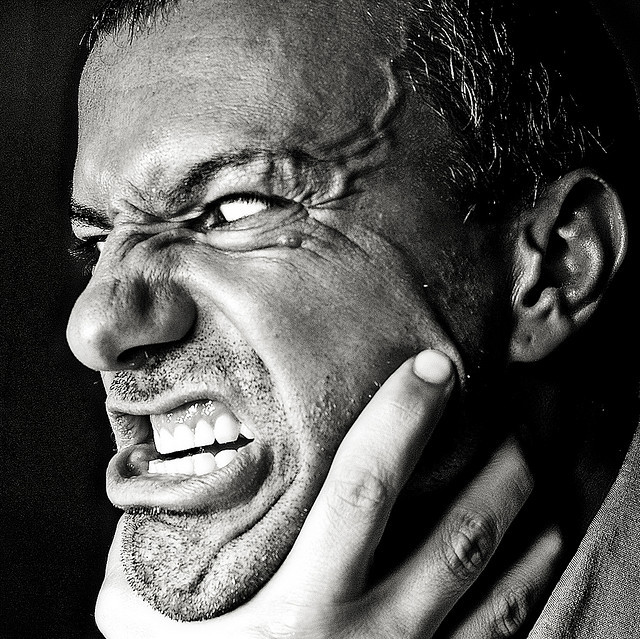
What kinds of studies would be necessary to assess these hypotheses? It is usually difficult to separate out the influences of environment and physiology on the development of behavior in humans. For example, boys and girls differ in their rough-and-tumble play at a very young age, which suggests an early physiological influence on aggression. However, parents interact with their male and female offspring differently; they usually play more roughly with male infants than with females, which suggests that the sex difference in aggressiveness is partially learned. This difference in parental interaction style is evident by the first week of life. Because of these complexities in the factors influencing human behavior, the study of hormonal effects on sex-differentiated behavior has been pursued in nonhuman animals, for which environmental influences can be held relatively constant. Animal models for which sexual differentiation occurs postnatally are often used so that this process can be easily manipulated experimentally.
Again, with the appropriate animal model, we can address the questions posed above: Is the sex difference in aggression due to higher adult blood concentrations of androgens in males than in females, or are males more aggressive than females because their brains are organized differently by perinatal hormones? Are males usually more aggressive than females because of an interaction of early and current blood androgen concentrations? If male mice are castrated prior to their sixth day of life, then treated with testosterone propionate in adulthood, they show low levels of aggression. Similarly, females ovariectomized prior to their sixth day but given androgens in adulthood do not express male-like levels of aggression. Treatment of perinatally gonadectomized males or females with testosterone prior to their sixth day life and also in adulthood results in a level of aggression similar to that observed in typical male mice. Thus, in mice, the proclivity for males to act more aggressively than females is organized perinatally by androgens but also requires the presence of androgens after puberty in order to be fully expressed. In other words, aggression in male mice is both organized and activated by androgens. Testosterone exposure in adulthood without prior organization of the brain by steroid hormones does not evoke typical male levels of aggression. The hormonal control of aggressive behavior in house mice is thus similar to the hormonal mediation of heterosexual male mating behavior in other rodent species. Aggressive behavior is both organized and activated by androgens in many species, including rats, hamsters, voles, dogs, and possibly some primate species.
Parental Behaviors
Parental behavior can be considered to be any behavior that contributes directly to the survival of fertilized eggs or offspring that have left the body of the female. There are many patterns of mammalian parental care. The developmental status of the newborn is an important factor driving the type and quality of parental care in a species. Maternal care is much more common than paternal care. The vast majority of research on the hormonal correlates of mammalian parental behavior has been conducted on rats. Rats bear altricial young, and mothers perform a cluster of stereotyped maternal behaviors, including nest building, crouching over the pups to allow nursing and to provide warmth, pup retrieval, and increased aggression directed at intruders. If you expose nonpregnant female rats (or males) to pups, their most common reaction is to huddle far away from them. Rats avoid new things (neophobia). However, if you expose adult rats to pups every day, they soon begin to behave maternally. This process is called concaveation or sensitization and it appears to serve to reduce the adult rats’ fear of pups.
Of course a new mother needs to act maternal as soon as her offspring arrive—not in a week. The onset of maternal behavior in rats is mediated by hormones. Several methods of study, such as hormone removal and replacement therapy, have been used to determine the hormonal correlates of rat maternal behavior. A fast decline of blood concentrations of progesterone in late pregnancy after sustained high concentrations of this hormone, in combination with high concentrations of estradiol and probably prolactin and oxytocin, induces female rats to behave maternally almost immediately in the presence of pups. This pattern of hormones at parturition overrides the usual fear response of adult rats toward pups, and it permits the onset of maternal behavior. Thus, the so-called maternal “instinct” requires hormones to increase the approach tendency and lower the avoidance tendency. Laboratory strains of mice and rats are usually docile, but mothers can be quite aggressive toward animals that venture too close to their litter. Progesterone appears to be the primary hormone that induces this maternal aggression in rodents, but species differences exist. The role of maternal aggression in women’s behavior has not been adequately described or tested.
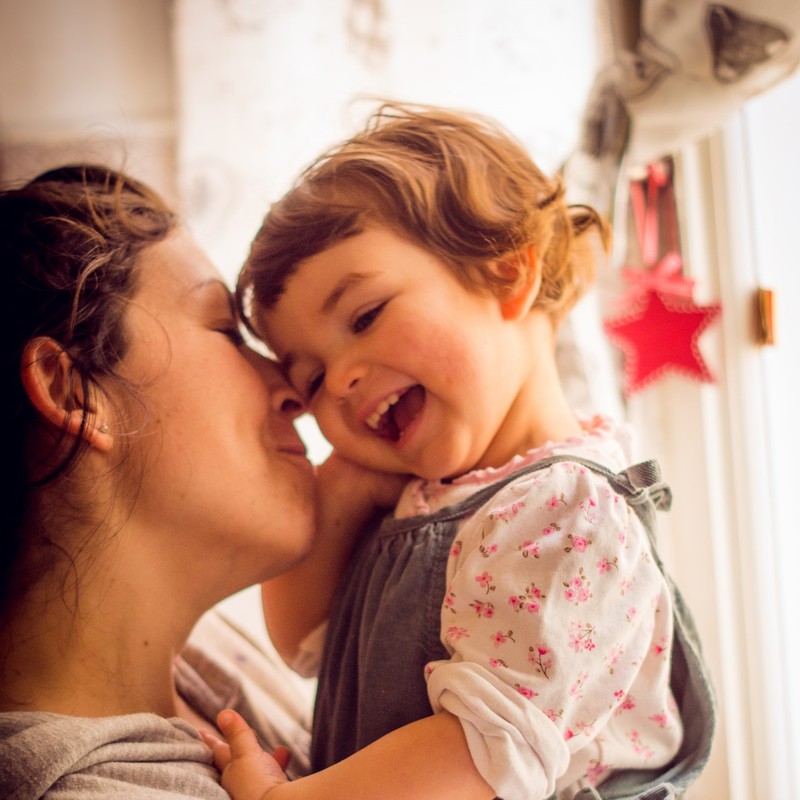
A series of elegant experiments by Alison Fleming and her collaborators studied the endocrine correlates of the behavior of human mothers as well as the endocrine correlates of maternal attitudes as expressed in self-report questionnaires. Responses such as patting, cuddling, or kissing the baby were called affectionate behaviors; talking, singing, or cooing to the baby were considered vocal behaviors. Both affectionate and vocal behaviors were considered approach behaviors. Basic caregiving activities, such as changing diapers and burping the infants, were also recorded. In these studies, no relationship between hormone concentrations and maternal responsiveness, as measured by attitude questionnaires, was found. For example, most women showed an increasing positive self-image during early pregnancy that dipped during the second half of pregnancy, but recovered after parturition. A related dip in feelings of maternal engagement occurred during late pregnancy, but rebounded substantially after birth in most women. However, when behavior, rather than questionnaire responses, was compared with hormone concentrations, a different story emerged. Blood plasma concentrations of cortisol were positively associated with approach behaviors. In other words, women who had high concentrations of blood cortisol, in samples obtained immediately before or after nursing, engaged in more physically affectionate behaviors and talked more often to their babies than mothers with low cortisol concentrations. Additional analyses from this study revealed that the correlation was even greater for mothers that had reported positive maternal regard (feelings and attitudes) during gestation. Indeed, nearly half of the variation in maternal behavior among women could be accounted for by cortisol concentrations and positive maternal attitudes during pregnancy.
Presumably, cortisol does not induce maternal behaviors directly, but it may act indirectly on the quality of maternal care by evoking an increase in the mother’s general level of arousal, thus increasing her responsiveness to infant-generated cues. New mothers with high cortisol concentrations were also more attracted to their infant’s odors, were superior in identifying their infants, and generally found cues from infants highly appealing (Fleming, Steiner, & Corter, 1997).
The medial preoptic area is critical for the expression of rat maternal behavior. The amygdala appears to tonically inhibit the expression of maternal behavior. Adult rats are fearful of pups, a response that is apparently mediated by chemosensory information. Lesions of the amygdala or afferent sensory pathways from the vomeronasal organ to the amygdala disinhibit the expression of maternal behavior. Hormones or sensitization likely act to disinhibit the amygdala, thus permitting the occurrence of maternal behavior. Although correlations have been established, direct evidence of brain structural changes in human mothers remains unspecified (Fleming & Gonzalez, 2009).
Considered together, there are many examples of hormones influencing behavior and of behavior feeding back to influence hormone secretion. More and more examples of hormone–behavior interactions are discovered, including hormones in the mediation of food and fluid intake, social interactions, salt balance, learning and memory, stress coping, as well as psychopathology including depression, anxiety disorders, eating disorders, postpartum depression, and seasonal depression. Additional research should reveal how these hormone–behavior interactions are mediated.
The Nature-Nurture Question
By Eric Turkheimer
University of Virginia
People have a deep intuition about what has been called the “nature–nurture question.” Some aspects of our behavior feel as though they originate in our genetic makeup, while others feel like the result of our upbringing or our own hard work. The scientific field of behavior genetics attempts to study these differences empirically, either by examining similarities among family members with different degrees of genetic relatedness, or, more recently, by studying differences in the DNA of people with different behavioral traits. The scientific methods that have been developed are ingenious, but often inconclusive. Many of the difficulties encountered in the empirical science of behavior genetics turn out to be conceptual, and our intuitions about nature and nurture get more complicated the harder we think about them. In the end, it is an oversimplification to ask how “genetic” some particular behavior is. Genes and environments always combine to produce behavior, and the real science is in the discovery of how they combine for a given behavior.
Learning Objectives
- Understand what the nature–nurture debate is and why the problem fascinates us.
- Understand why nature–nurture questions are difficult to study empirically.
- Know the major research designs that can be used to study nature–nurture questions.
- Appreciate the complexities of nature–nurture and why questions that seem simple turn out not to have simple answers.
Introduction
There are three related problems at the intersection of philosophy and science that are fundamental to our understanding of our relationship to the natural world: the mind–body problem, the free will problem, and the nature–nurture problem. These great questions have a lot in common. Everyone, even those without much knowledge of science or philosophy, has opinions about the answers to these questions that come simply from observing the world we live in. Our feelings about our relationship with the physical and biological world often seem incomplete. We are in control of our actions in some ways, but at the mercy of our bodies in others; it feels obvious that our consciousness is some kind of creation of our physical brains, at the same time we sense that our awareness must go beyond just the physical. This incomplete knowledge of our relationship with nature leaves us fascinated and a little obsessed, like a cat that climbs into a paper bag and then out again, over and over, mystified every time by a relationship between inner and outer that it can see but can’t quite understand.
It may seem obvious that we are born with certain characteristics while others are acquired, and yet of the three great questions about humans’ relationship with the natural world, only nature–nurture gets referred to as a “debate.” In the history of psychology, no other question has caused so much controversy and offense: We are so concerned with nature–nurture because our very sense of moral character seems to depend on it. While we may admire the athletic skills of a great basketball player, we think of his height as simply a gift, a payoff in the “genetic lottery.” For the same reason, no one blames a short person for his height or someone’s congenital disability on poor decisions: To state the obvious, it’s “not their fault.” But we do praise the concert violinist (and perhaps her parents and teachers as well) for her dedication, just as we condemn cheaters, slackers, and bullies for their bad behavior.
The problem is, most human characteristics aren’t usually as clear-cut as height or instrument-mastery, affirming our nature–nurture expectations strongly one way or the other. In fact, even the great violinist might have some inborn qualities—perfect pitch, or long, nimble fingers—that support and reward her hard work. And the basketball player might have eaten a diet while growing up that promoted his genetic tendency for being tall. When we think about our own qualities, they seem under our control in some respects, yet beyond our control in others. And often the traits that don’t seem to have an obvious cause are the ones that concern us the most and are far more personally significant. What about how much we drink or worry? What about our honesty, or religiosity, or sexual orientation? They all come from that uncertain zone, neither fixed by nature nor totally under our own control.
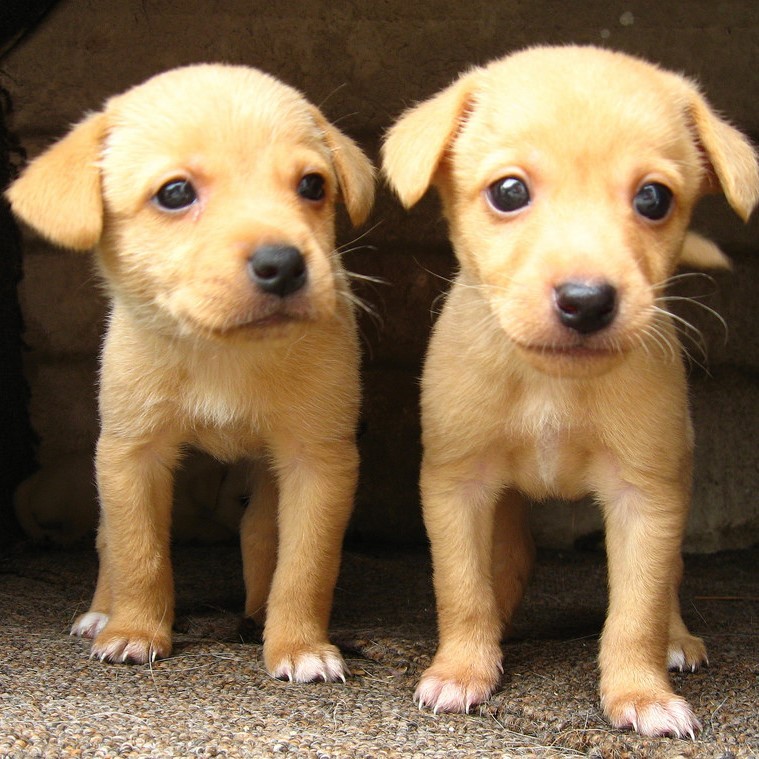
One major problem with answering nature-nurture questions about people is, how do you set up an experiment? In nonhuman animals, there are relatively straightforward experiments for tackling nature–nurture questions. Say, for example, you are interested in aggressiveness in dogs. You want to test for the more important determinant of aggression: being born to aggressive dogs or being raised by them. You could mate two aggressive dogs—angry Chihuahuas—together, and mate two nonaggressive dogs—happy beagles—together, then switch half the puppies from each litter between the different sets of parents to raise. You would then have puppies born to aggressive parents (the Chihuahuas) but being raised by nonaggressive parents (the Beagles), and vice versa, in litters that mirror each other in puppy distribution. The big questions are: Would the Chihuahua parents raise aggressive beagle puppies? Would the beagle parents raise nonaggressive Chihuahua puppies? Would the puppies’ nature win out, regardless of who raised them? Or… would the result be a combination of nature and nurture? Much of the most significant nature–nurture research has been done in this way (Scott & Fuller, 1998), and animal breeders have been doing it successfully for thousands of years. In fact, it is fairly easy to breed animals for behavioral traits.
With people, however, we can’t assign babies to parents at random, or select parents with certain behavioral characteristics to mate, merely in the interest of science (though history does include horrific examples of such practices, in misguided attempts at “eugenics,” the shaping of human characteristics through intentional breeding). In typical human families, children’s biological parents raise them, so it is very difficult to know whether children act like their parents due to genetic (nature) or environmental (nurture) reasons. Nevertheless, despite our restrictions on setting up human-based experiments, we do see real-world examples of nature-nurture at work in the human sphere—though they only provide partial answers to our many questions.
The science of how genes and environments work together to influence behavior is called behavioral genetics. The easiest opportunity we have to observe this is the adoption study. When children are put up for adoption, the parents who give birth to them are no longer the parents who raise them. This setup isn’t quite the same as the experiments with dogs (children aren’t assigned to random adoptive parents in order to suit the particular interests of a scientist) but adoption still tells us some interesting things, or at least confirms some basic expectations. For instance, if the biological child of tall parents were adopted into a family of short people, do you suppose the child’s growth would be affected? What about the biological child of a Spanish-speaking family adopted at birth into an English-speaking family? What language would you expect the child to speak? And what might these outcomes tell you about the difference between height and language in terms of nature-nurture?
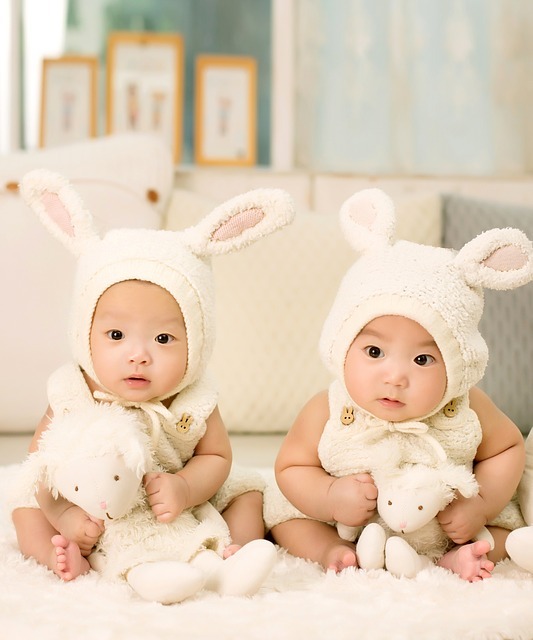
Another option for observing nature-nurture in humans involves twin studies. There are two types of twins: monozygotic (MZ) and dizygotic (DZ). Monozygotic twins, also called “identical” twins, result from a single zygote (fertilized egg) and have the same DNA. They are essentially clones. Dizygotic twins, also known as “fraternal” twins, develop from two zygotes and share 50% of their DNA. Fraternal twins are ordinary siblings who happen to have been born at the same time. To analyze nature–nurture using twins, we compare the similarity of MZ and DZ pairs. Sticking with the features of height and spoken language, let’s take a look at how nature and nurture apply: Identical twins, unsurprisingly, are almost perfectly similar for height. The heights of fraternal twins, however, are like any other sibling pairs: more similar to each other than to people from other families, but hardly identical. This contrast between twin types gives us a clue about the role genetics plays in determining height. Now consider spoken language. If one identical twin speaks Spanish at home, the co-twin with whom she is raised almost certainly does too. But the same would be true for a pair of fraternal twins raised together. In terms of spoken language, fraternal twins are just as similar as identical twins, so it appears that the genetic match of identical twins doesn’t make much difference.
Twin and adoption studies are two instances of a much broader class of methods for observing nature-nurture called quantitative genetics, the scientific discipline in which similarities among individuals are analyzed based on how biologically related they are. We can do these studies with siblings and half-siblings, cousins, twins who have been separated at birth and raised separately (Bouchard, Lykken, McGue, & Segal, 1990; such twins are very rare and play a smaller role than is commonly believed in the science of nature–nurture), or with entire extended families (see Plomin, DeFries, Knopik, & Neiderhiser, 2012, for a complete introduction to research methods relevant to nature–nurture).
For better or for worse, contentions about nature–nurture have intensified because quantitative genetics produces a number called a heritability coefficient, varying from 0 to 1, that is meant to provide a single measure of genetics’ influence of a trait. In a general way, a heritability coefficient measures how strongly differences among individuals are related to differences among their genes. But beware: Heritability coefficients, although simple to compute, are deceptively difficult to interpret. Nevertheless, numbers that provide simple answers to complicated questions tend to have a strong influence on the human imagination, and a great deal of time has been spent discussing whether the heritability of intelligence or personality or depression is equal to one number or another.
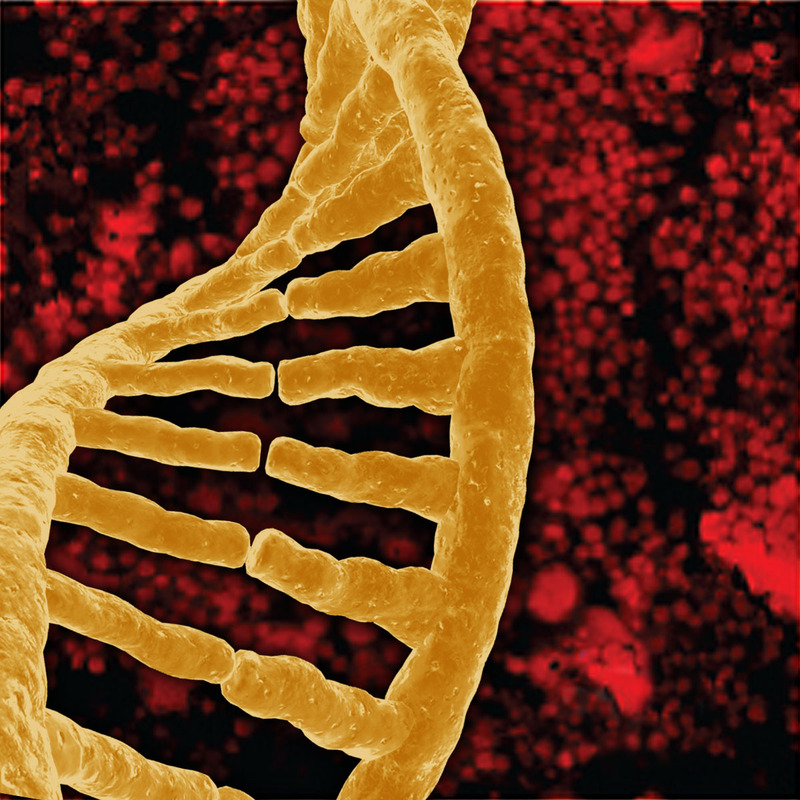
One reason nature–nurture continues to fascinate us so much is that we live in an era of great scientific discovery in genetics, comparable to the times of Copernicus, Galileo, and Newton, with regard to astronomy and physics. Every day, it seems, new discoveries are made, new possibilities proposed. When Francis Galton first started thinking about nature–nurture in the late-19th century he was very influenced by his cousin, Charles Darwin, but genetics per se was unknown. Mendel’s famous work with peas, conducted at about the same time, went undiscovered for 20 years; quantitative genetics was developed in the 1920s; DNA was discovered by Watson and Crick in the 1950s; the human genome was completely sequenced at the turn of the 21st century; and we are now on the verge of being able to obtain the specific DNA sequence of anyone at a relatively low cost. No one knows what this new genetic knowledge will mean for the study of nature–nurture, but as we will see in the next section, answers to nature–nurture questions have turned out to be far more difficult and mysterious than anyone imagined.
What Have We Learned About Nature–Nurture?
It would be satisfying to be able to say that nature–nurture studies have given us conclusive and complete evidence about where traits come from, with some traits clearly resulting from genetics and others almost entirely from environmental factors, such as childrearing practices and personal will; but that is not the case. Instead, everything has turned out to have some footing in genetics. The more genetically-related people are, the more similar they are—for everything: height, weight, intelligence, personality, mental illness, etc. Sure, it seems like common sense that some traits have a genetic bias. For example, adopted children resemble their biological parents even if they have never met them, and identical twins are more similar to each other than are fraternal twins. And while certain psychological traits, such as personality or mental illness (e.g., schizophrenia), seem reasonably influenced by genetics, it turns out that the same is true for political attitudes, how much television people watch (Plomin, Corley, DeFries, & Fulker, 1990), and whether or not they get divorced (McGue & Lykken, 1992).
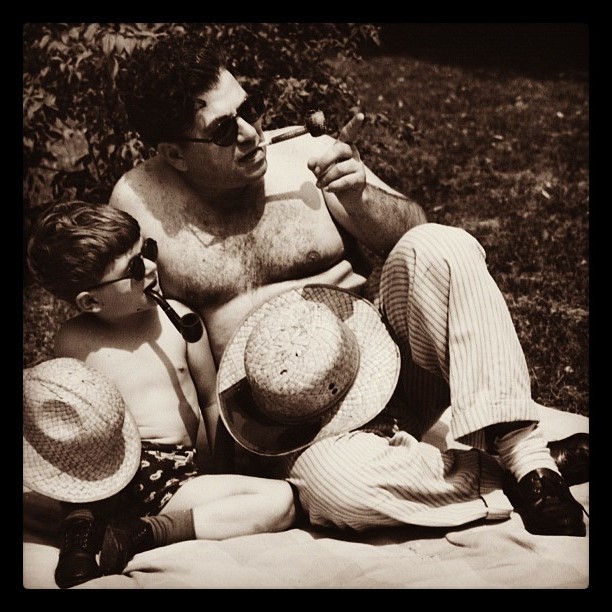
It may seem surprising, but genetic influence on behavior is a relatively recent discovery. In the middle of the 20th century, psychology was dominated by the doctrine of behaviorism, which held that behavior could only be explained in terms of environmental factors. Psychiatry concentrated on psychoanalysis, which probed for roots of behavior in individuals’ early life-histories. The truth is, neither behaviorism nor psychoanalysis is incompatible with genetic influences on behavior, and neither Freud nor Skinner was naive about the importance of organic processes in behavior. Nevertheless, in their day it was widely thought that children’s personalities were shaped entirely by imitating their parents’ behavior, and that schizophrenia was caused by certain kinds of “pathological mothering.” Whatever the outcome of our broader discussion of nature–nurture, the basic fact that the best predictors of an adopted child’s personality or mental health are found in the biological parents he or she has never met, rather than in the adoptive parents who raised him or her, presents a significant challenge to purely environmental explanations of personality or psychopathology. The message is clear: You can’t leave genes out of the equation. But keep in mind, no behavioral traits are completely inherited, so you can’t leave the environment out altogether, either.
Trying to untangle the various ways nature-nurture influences human behavior can be messy, and often common-sense notions can get in the way of good science. One very significant contribution of behavioral genetics that has changed psychology for good can be very helpful to keep in mind: When your subjects are biologically-related, no matter how clearly a situation may seem to point to environmental influence, it is never safe to interpret a behavior as wholly the result of nurture without further evidence. For example, when presented with data showing that children whose mothers read to them often are likely to have better reading scores in third grade, it is tempting to conclude that reading to your kids out loud is important to success in school; this may well be true, but the study as described is inconclusive, because there are genetic as well asenvironmental pathways between the parenting practices of mothers and the abilities of their children. This is a case where “correlation does not imply causation,” as they say. To establish that reading aloud causes success, a scientist can either study the problem in adoptive families (in which the genetic pathway is absent) or by finding a way to randomly assign children to oral reading conditions.
The outcomes of nature–nurture studies have fallen short of our expectations (of establishing clear-cut bases for traits) in many ways. The most disappointing outcome has been the inability to organize traits from more– to less-genetic. As noted earlier, everything has turned out to be at least somewhat heritable (passed down), yet nothing has turned out to be absolutely heritable, and there hasn’t been much consistency as to which traits are moreheritable and which are less heritable once other considerations (such as how accurately the trait can be measured) are taken into account (Turkheimer, 2000). The problem is conceptual: The heritability coefficient, and, in fact, the whole quantitative structure that underlies it, does not match up with our nature–nurture intuitions. We want to know how “important” the roles of genes and environment are to the development of a trait, but in focusing on “important” maybe we’re emphasizing the wrong thing. First of all, genes and environment are both crucial to every trait; without genes the environment would have nothing to work on, and too, genes cannot develop in a vacuum. Even more important, because nature–nurture questions look at the differences among people, the cause of a given trait depends not only on the trait itself, but also on the differences in that trait between members of the group being studied.
The classic example of the heritability coefficient defying intuition is the trait of having two arms. No one would argue against the development of arms being a biological, genetic process. But fraternal twins are just as similar for “two-armedness” as identical twins, resulting in a heritability coefficient of zero for the trait of having two arms. Normally, according to the heritability model, this result (coefficient of zero) would suggest all nurture, no nature, but we know that’s not the case. The reason this result is not a tip-off that arm development is less genetic than we imagine is because people do not vary in the genes related to arm development—which essentially upends the heritability formula. In fact, in this instance, the opposite is likely true: the extent that people differ in arm number is likely the result of accidents and, therefore, environmental. For reasons like these, we always have to be very careful when asking nature–nurture questions, especially when we try to express the answer in terms of a single number. The heritability of a trait is not simply a property of that trait, but a property of the trait in a particular context of relevant genes and environmental factors.
Another issue with the heritability coefficient is that it divides traits’ determinants into two portions—genes and environment—which are then calculated together for the total variability. This is a little like asking how much of the experience of a symphony comes from the horns and how much from the strings; the ways instruments or genes integrate is more complex than that. It turns out to be the case that, for many traits, genetic differences affect behavior under some environmental circumstances but not others—a phenomenon called gene-environment interaction, or G x E. In one well-known example, Caspi et al. (2002) showed that among maltreated children, those who carried a particular allele of the MAOA gene showed a predisposition to violence and antisocial behavior, while those with other alleles did not. Whereas, in children who had not been maltreated, the gene had no effect. Making matters even more complicated are very recent studies of what is known as epigenetics (see module, “Epigenetics” http://noba.to/37p5cb8v), a process in which the DNA itself is modified by environmental events, and those genetic changes transmitted to children.
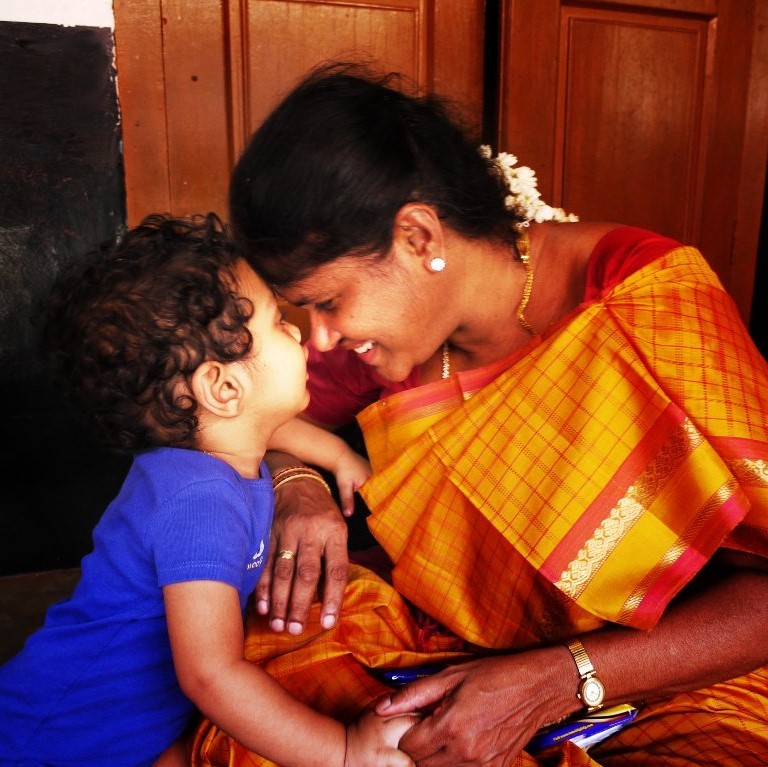
Some common questions about nature–nurture are, how susceptible is a trait to change, how malleable is it, and do we “have a choice” about it? These questions are much more complex than they may seem at first glance. For example, phenylketonuria is an inborn error of metabolism caused by a single gene; it prevents the body from metabolizing phenylalanine. Untreated, it causes mental retardation and death. But it can be treated effectively by a straightforward environmental intervention: avoiding foods containing phenylalanine. Height seems like a trait firmly rooted in our nature and unchangeable, but the average height of many populations in Asia and Europe has increased significantly in the past 100 years, due to changes in diet and the alleviation of poverty. Even the most modern genetics has not provided definitive answers to nature–nurture questions. When it was first becoming possible to measure the DNA sequences of individual people, it was widely thought that we would quickly progress to finding the specific genes that account for behavioral characteristics, but that hasn’t happened. There are a few rare genes that have been found to have significant (almost always negative) effects, such as the single gene that causes Huntington’s disease, or the Apolipoprotein gene that causes early onset dementia in a small percentage of Alzheimer’s cases. Aside from these rare genes of great effect, however, the genetic impact on behavior is broken up over many genes, each with very small effects. For most behavioral traits, the effects are so small and distributed across so many genes that we have not been able to catalog them in a meaningful way. In fact, the same is true of environmental effects. We know that extreme environmental hardship causes catastrophic effects for many behavioral outcomes, but fortunately extreme environmental hardship is very rare. Within the normal range of environmental events, those responsible for differences (e.g., why some children in a suburban third-grade classroom perform better than others) are much more difficult to grasp.
The difficulties with finding clear-cut solutions to nature–nurture problems bring us back to the other great questions about our relationship with the natural world: the mind-body problem and free will. Investigations into what we mean when we say we are aware of something reveal that consciousness is not simply the product of a particular area of the brain, nor does choice turn out to be an orderly activity that we can apply to some behaviors but not others. So it is with nature and nurture: What at first may seem to be a straightforward matter, able to be indexed with a single number, becomes more and more complicated the closer we look. The many questions we can ask about the intersection among genes, environments, and human traits—how sensitive are traits to environmental change, and how common are those influential environments; are parents or culture more relevant; how sensitive are traits to differences in genes, and how much do the relevant genes vary in a particular population; does the trait involve a single gene or a great many genes; is the trait more easily described in genetic or more-complex behavioral terms?—may have different answers, and the answer to one tells us little about the answers to the others.
It is tempting to predict that the more we understand the wide-ranging effects of genetic differences on all human characteristics—especially behavioral ones—our cultural, ethical, legal, and personal ways of thinking about ourselves will have to undergo profound changes in response. Perhaps criminal proceedings will consider genetic background. Parents, presented with the genetic sequence of their children, will be faced with difficult decisions about reproduction. These hopes or fears are often exaggerated. In some ways, our thinking may need to change—for example, when we consider the meaning behind the fundamental American principle that all men are created equal. Human beings differ, and like all evolved organisms they differ genetically. The Declaration of Independence predates Darwin and Mendel, but it is hard to imagine that Jefferson—whose genius encompassed botany as well as moral philosophy—would have been alarmed to learn about the genetic diversity of organisms. One of the most important things modern genetics has taught us is that almost all human behavior is too complex to be nailed down, even from the most complete genetic information, unless we’re looking at identical twins. The science of nature and nurture has demonstrated that genetic differences among people are vital to human moral equality, freedom, and self-determination, not opposed to them. As Mordecai Kaplan said about the role of the past in Jewish theology, genetics gets a vote, not a veto, in the determination of human behavior. We should indulge our fascination with nature–nurture while resisting the temptation to oversimplify it.
Outside Resources
- Book: Adkins-Regan, E. (2005). Hormones and animal social behavior. Princeton, NJ: Princeton University Press.
- Book: Beach, F. A. (1948). Hormones and behavior. New York: Paul Hoeber.
- Book: Beach, F. A. (1975). Behavioral endocrinology: An emerging discipline. American Scientist, 63: 178–187.
- Book: Nelson, R. J. (2011). An introduction to behavioral endocrinology (4th ed.). Sunderland, MA: Sinauer Associates.
- Book: Pfaff, D. W. (2009). Hormones, brain, and behavior (2nd ed.). New York: Academic Press.
- Book: Pfaff, D. W., Phillips, I. M., & Rubin, R. T. (2005). Principles of hormone/behavior relations. New York: Academic Press.
- Video: Endocrinology Video (Playlist) – This YouTube playlist contains many helpful videos on the biology of hormones, including reproduction and behavior. This would be a helpful resource for students struggling with hormone synthesis, reproduction, regulation of biological functions, and signaling pathways.
- https://www.youtube.com/playlist?list=PLqTetbgey0aemiTfD8QkMsSUq8hQzv-vA
- Web: Society for Behavioral Neuroendocrinology – This website contains resources on current news and research in the field of neuroendocrinology.
- http://sbn.org/home.aspx
- Web: Institute for Behavioral Genetics
Discussion Questions
- What are some of the problems associated with attempting to determine causation in a hormone–behavior interaction? What are the best ways to address these problems?
- Hormones cause changes in the rates of cellular processes or in cellular morphology. What are some ways that these hormonally induced cellular changes might theoretically produce profound changes in behavior?
- List and describe some behavioral sex differences that you have noticed between boys and girls. What causes girls and boys to choose different toys? Do you think that the sex differences you have noted arise from biological causes or are learned? How would you go about establishing your opinions as fact?
- Why is it inappropriate to refer to androgens as “male” hormones and estrogens as “female” hormones?
- Imagine that you discovered that the brains of architects were different from those of non-architects—specifically, that the “drawstraightem nuclei” of the right temporal lobe were enlarged in architects as compared with non-architects. Would you argue that architects were destined to be architects because of their brain organization or that experience as an architect changed their brains? How would you resolve this issue?
- Is your personality more like one of your parents than the other? If you have a sibling, is his or her personality like yours? In your family, how did these similarities and differences develop? What do you think caused them?
- Can you think of a human characteristic for which genetic differences would play almost no role? Defend your choice.
- Do you think the time will come when we will be able to predict almost everything about someone by examining their DNA on the day they are born?
- Identical twins are more similar than fraternal twins for the trait of aggressiveness, as well as for criminal behavior. Do these facts have implications for the courtroom? If it can be shown that a violent criminal had violent parents, should it make a difference in culpability or sentencing?
Vocabulary
- 5α-reductase
- An enzyme required to convert testosterone to 5α-dihydrotestosterone.
- Aggression
- A form of social interaction that includes threat, attack, and fighting.
- Aromatase
- An enzyme that converts androgens into estrogens.
- Chromosomal sex
- The sex of an individual as determined by the sex chromosomes (typically XX or XY) received at the time of fertilization.
- Defeminization
- The removal of the potential for female traits.
- Demasculinization
- The removal of the potential for male traits.
- Dihydrotestosterone (DHT)
- A primary androgen that is an androgenic steroid product of testosterone and binds strongly to androgen receptors.
- Endocrine gland
- A ductless gland from which hormones are released into the blood system in response to specific biological signals.
- Estrogen
- Any of the C18 class of steroid hormones, so named because of the estrus-generating properties in females. Biologically important estrogens include estradiol and estriol.
- Feminization
- The induction of female traits.
- Gonadal sex
- The sex of an individual as determined by the possession of either ovaries or testes. Females have ovaries, whereas males have testes.
- Hormone
- An organic chemical messenger released from endocrine cells that travels through the blood to interact with target cells at some distance to cause a biological response.
- Masculinization
- The induction of male traits.
- Maternal behavior
- Parental behavior performed by the mother or other female.
- Neurotransmitter
- A chemical messenger that travels between neurons to provide communication. Some neurotransmitters, such as norepinephrine, can leak into the blood system and act as hormones.
- Oxytocin
- A peptide hormone secreted by the pituitary gland to trigger lactation, as well as social bonding.
- Parental behavior
- Behaviors performed in relation to one’s offspring that contributes directly to the survival of those offspring
- Paternal behavior
- Parental behavior performed by the father or other male.
- Progesterone
- A primary progestin that is involved in pregnancy and mating behaviors.
- Progestin
- A class of C21 steroid hormones named for their progestational (pregnancy-supporting) effects. Progesterone is a common progestin.
- Prohormone
- A molecule that can act as a hormone itself or be converted into another hormone with different properties. For example, testosterone can serve as a hormone or as a prohormone for either dihydrotestosterone or estradiol.
- Prolactin
- A protein hormone that is highly conserved throughout the animal kingdom. It has many biological functions associated with reproduction and synergistic actions with steroid hormones.
- Receptor
- A chemical structure on the cell surface or inside of a cell that has an affinity for a specific chemical configuration of a hormone, neurotransmitter, or other compound.
- Sex determination
- The point at which an individual begins to develop as either a male or a female. In animals that have sex chromosomes, this occurs at fertilization. Females are XX and males are XY. All eggs bear X chromosomes, whereas sperm can either bear X or Y chromosomes. Thus, it is the males that determine the sex of the offspring.
- Sex differentiation
- The process by which individuals develop the characteristics associated with being male or female. Differential exposure to gonadal steroids during early development causes sexual differentiation of several structures including the brain.
- Target cell
- A cell that has receptors for a specific chemical messenger (hormone or neurotransmitter).
- Testosterone
- The primary androgen secreted by the testes of most vertebrate animals, including men.
-
- Adoption study
- A behavior genetic research method that involves comparison of adopted children to their adoptive and biological parents.
- Behavioral genetics
- The empirical science of how genes and environments combine to generate behavior.
- Heritability coefficient
- An easily misinterpreted statistical construct that purports to measure the role of genetics in the explanation of differences among individuals.
- Quantitative genetics
- Scientific and mathematical methods for inferring genetic and environmental processes based on the degree of genetic and environmental similarity among organisms.
- Twin studies
- A behavior genetic research method that involves comparison of the similarity of identical (monozygotic; MZ) and fraternal (dizygotic; DZ) twins.
References
- Alexander, G. M. & Hines, M. (2002). Sex differences in response to children’s toys in nonhuman primates (Cercopithecus aethiops sabaeus). Evolution and Human Behavior, 23, 467–479.
- Berenbaum, S. A., Martin, C. L., Hanish, L. D., Briggs, P. T., & Fabes, R. A. (2008). Sex differences in children’s play. In J. B. Becker, K. J. Berkley, N. Geary, E. Hampson, J. Herman, & E. Young (Eds.), Sex differences in the brain: From genes to behavior. New York: Oxford University Press.
- Dabbs, J. M. (2000). Heroes, rogues, and lovers: Testosterone and behavior. Columbus, OH: McGraw Hill.
- Fleming, A. S., & Gonzalez, A. (2009). Neurobiology of human maternal care. In P. T. Ellison & P. B. Gray (Eds.), Endocrinology of social relationships (pp. 294–318). Cambridge, MA: Harvard University Press.
- Fleming, A. S., Steiner, M., & Corter, C. (1997). Cortisol, hedonics, and maternal responsiveness in human mothers. Hormones and Behavior, 32, 85–98.
- Goodson, J. L., Saldanha, C. J., Hahn, T. P., Soma, K. K. (2005). Recent advances in behavioral neuroendocrinology: Insights from studies on birds. Hormones and Behavior, 48, 461–73.
- Kidd, K. A., Blanchfield, P. J., Mills, K. H., Palace, V. P., Evans, R. E. Lazorchak, J. M. & Flick, R. (2007). Collapse of a fish population following exposure to a synthetic estrogen. Proceedings of the National Academy of Sciences,104, 8897–8901.
- Nelson, R. J. (Ed.) (2006). Biology of aggression. New York: Oxford University Press.
- Nelson, R.J. (2011). An introduction to behavioral endocrinology (4th ed.). Sunderland, MA: Sinauer Associates.
- Phoenix, C. H., Goy, R. W., Gerall, A. A., & Young, W. C. (1959). Organizing action of prenatally administered testosterone propionate on the tissues mediating mating behavior in the female guinea pig. Endocrinology, 65: 369–382.
- van Anders, S., Hamilton, L., Schmidt, N., & Watson, N. (2007). Associations between testosterone secretion and sexual activity in women. Hormones and Behavior, 51, 477–82.
- Bouchard, T. J., Lykken, D. T., McGue, M., & Segal, N. L. (1990). Sources of human psychological differences: The Minnesota study of twins reared apart. Science, 250(4978), 223–228.
- Caspi, A., McClay, J., Moffitt, T. E., Mill, J., Martin, J., Craig, I. W., Taylor, A. & Poulton, R. (2002). Role of genotype in the cycle of violence in maltreated children. Science, 297(5582), 851–854.
- McGue, M., & Lykken, D. T. (1992). Genetic influence on risk of divorce. Psychological Science, 3(6), 368–373.
- Plomin, R., Corley, R., DeFries, J. C., & Fulker, D. W. (1990). Individual differences in television viewing in early childhood: Nature as well as nurture. Psychological Science, 1(6), 371–377.
- Plomin, R., DeFries, J. C., Knopik, V. S., & Neiderhiser, J. M. (2012). Behavioral genetics. New York, NY: Worth Publishers.
- Scott, J. P., & Fuller, J. L. (1998). Genetics and the social behavior of the dog. Chicago, IL: University of Chicago Press.
- Turkheimer, E. (2000). Three laws of behavior genetics and what they mean. Current Directions in Psychological Science, 9(5), 160–164.
Authors
Randy J. Nelson has two PhDs (Psychology and Endocrinology) and is Professor and Chair of Neuroscience and Professor of Psychology at The Ohio State University. He has been appointed as University Distinguished Professor for his work on hormones, biological rhythms, and behavior. Dr. Nelson has published nearly 400 papers and several books on these topics.
Eric Turkheimer is a Professor of Psychology at the University of Virginia. Â He was President of the Behavior Genetics Association in 2012-2013. Â He won the James Shields Award for Twin Research in 2009. Â His work explores the possibilities and limitations of genetics research as a tool for understanding complex human behavior.
Creative Commons License
The Nature-Nurture Question by Eric Turkheimer is licensed under a Creative Commons Attribution-NonCommercial-ShareAlike 4.0 International License. Permissions beyond the scope of this license may be available in our Licensing Agreement.
Creative Commons License
Hormones & Behavior by Randy J. Nelson is licensed under a Creative Commons Attribution-NonCommercial-ShareAlike 4.0 International License. Permissions beyond the scope of this license may be available in our Licensing Agreement.